Investigation of Heat Transfer Characteristics and Electrical Conductivities in NaCl, KCl, and NaNO3 Solutions
DOI:
https://doi.org/10.46604/aiti.2024.14330Keywords:
heat transfer coefficient, conductivity, sodium chloride (NaCl), potassium chloride (KCl), sodium nitrate (NaNO3)Abstract
This study aims to optimize heat exchanger systems by investigating the effects of water-soluble salts (NaCl, KCl, and NaNO3) on heat transfer rates and electrical conductivity. Experiments are conducted using plate-type (PHE) and double-pipe (DPHE) heat exchangers. The heat transfer coefficient ranged from 1.5–6.5 kW/m²K in PHE and 3.5–20 kW/m²K in DPHE. NaCl achieves the highest heat transfer rates, followed by KCl and NaNO3, all outperforming pure water. Electrical conductivity peaks at 1 MHz, decreasing afterward, with NaCl and KCl showing higher conductivity than NaNO3. Conductivity increases with temperature, peaking at 70°C, and is more sensitive to temperature for KCl and NaCl. This dual-focus study correlates thermal and electrical properties, illustrating how variations in salt type, concentration, and temperature influence ion behavior, which plays a critical role in optimizing industrial heat transfer and electrical conductivity processes.
References
A. F. Faraj, I. D. J. Azzawi, and S. G. Yahya, “Pitch Variations Study on Helically Coiled Pipe in Turbulent Flow Region Using CFD,” International Journal of Heat and Technology, vol. 38, no. 4, pp. 775-784, 2020.
A. Al-damook and I. D. J. Azzawi, “Multi-objective Numerical Optimum Design of Natural Convection in Different Configurations of Concentric Horizontal Annular Pipes Using Different Nanofluids,” Heat and Mass Transfer, vol. 57, pp. 1543-1557, 2021.
M. Zaboli, S. S. M. Ajarostaghi, M. Noorbakhsh, and M. A. Delavar, “Effects of Geometrical and Operational Parameters on Heat Transfer and Fluid Flow of Three Various Water Based Nanofluids in a Shell and Coil Tube Heat Exchanger,” SN Applied Sciences, vol. 1, article no. 1387, 2019.
J. T. Cieśliński, A. Fiuk, K. Typiński, and B. Siemieńczuk, “Heat Transfer in Plate Heat Exchanger Channels: Experimental Validation of Selected Correlation Equations,” Archives of Thermodynamics, vol. 37, no. 3, pp. 19-29, 2016.
B. Kumar, A. Soni, and S. N. Singh, “Effect of Geometrical Parameters on the Performance of Chevron Type Plate Heat Exchanger,” Experimental Thermal and Fluid Science, vol. 91, Pages 126-133, 2018.
C. F. Cetinbas, B. A. Tuna, C. Akin, S. Aradag, and N. S. Uzol, “Comparison of Gasketed Plate Heat Exchangers with Double Pipe Heat Exchangers,” Proceedings of ASME 10th Biennial Conference on Engineering Systems Design and Analysis, vol. 2, pp. 643-651, 2010.
A. Najm, I. D. J. Azzawi, and A. M. A. Karim, “Experimental and Numerical Investigation of Heat Transfer Enhancement in Double Coil Heat Exchanger,” Journal of Thermal Engineering, vol. 10, no. 1, pp. 62-77, 2024.
S. Kakaç, H. Liu, and A. Pramuanjaroenkij, Heat Exchangers: Selection, Rating, and Thermal Design, 2nd ed., Florida: CRC Press, 2002.
W. M. Nagle, “Mean Temperature Differences in Multipass Heat Exchangers,” Industrial & Engineering Chemistry, vol. 25, no. 6, pp. 604-609, 1933.
S. J. M. Cartaxo and F. A. N. Fernandes, “Counterflow Logarithmic Mean Temperature Difference is Actually the Upper Bound: A Demonstration,” Applied Thermal Engineering, vol. 31, no. 6-7, pp. 1172-1175, 2011.
O. Drecun, A. Striolo, and C. Bernardini, “Structural and Dynamic Properties of Some Aqueous Salt Solutions,” Physical Chemistry Chemical Physics, vol. 23, no. 28, pp. 15224-15235, 2021.
S. Kakaç and A. Pramuanjaroenkij, “Review of Convective Heat Transfer Enhancement with Nanofluids,” International Journal of Heat and Mass Transfer, vol. 52, no. 13-14, pp. 3187-3196, 2009.
M. A. Adriana, “Hybrid Nanofluids Based on Al2O3, TiO2, and SiO2: Numerical Evaluation of Different Approaches,” International Journal of Heat and Mass Transfer, vol. 104, pp. 852-860, 2017.
A. Rehman, S. Ahmad, S. A. AlQahtani, M. Inc, S. Rezapour, and N. F. AlQahtani, et al., “Analytical Study of MHD Stagnation Point Flow with the Impact of Thermal Radiation and Viscous Dissipation Over Stretching Surface,” Modern Physics Letters B, vol. 39, no. 05, article no. 2450359, 2025.
W. Zhang, X. Chen, Y. Wang, L. Wu, and Y. Hu, “Experimental and Modeling of Conductivity for Electrolyte Solution Systems,” ACS Omega, vol. 5, no. 35, pp. 22465-22474, 2020.
A. Chandra and B. Bagchi, “Frequency Dependence of Ionic Conductivity of Electrolyte Solutions,” The Journal of Chemical Physics, vol. 112, pp. 1876-1886, 2000.
T. Yamaguchi, T. Matsuoka, and S. Koda, “A Theoretical Study on the Frequency-Dependent Electric Conductivity of Electrolyte Solutions. II. Effect of Hydrodynamic Interaction,” The Journal of Chemical Physics, vol. 130, article no. 094506, 2009.
R. Aghayari, H. Maddah, M. Zarei, M. Dehghani, and S. G. K. Mahalle, “Heat Transfer of Nanofluid in a Double Pipe Heat Exchanger,” International Scholarly Research Notices, vol. 2014, no. 1, article no. 736424, 2014.
B. Bakthavatchalam, K. Habib, R. Saidur, B. B. Saha, and K. Irshad, “Comprehensive Study on Nanofluid and Ionanofluid for Heat Transfer Enhancement: A Review on Current and Future Perspective,” Journal of Molecular Liquids, vol. 305, article no. 112787, 2020.
M. L. G. Ho, C. S. Oon, L. L. Tan, Y. Wang, and Y. M. Hung, “A Review on Nanofluids Coupled with Extended Surfaces for Heat Transfer Enhancement,” Results in Engineering, vol. 17, article no. 100957, 2023.
S. U. S. Choi, and J. A. Eastman, “Enhancing Thermal Conductivity of Fluids with Nanoparticles,” Proceedings of ASME International Mechanical Engineering Congress and Exposition, article no. 196525, 1995.
M. Laliberté, “A Model for Calculating the Heat Capacity of Aqueous Solutions, with Updated Density and Viscosity Data,” Journal of Chemical & Engineering Data, vol. 54, no. 6, pp. 1725-1760, 2009.
M. W. Chase, “NIST-JANAF Thermochemical Tables, 4th Edition,” https://www.nist.gov/publications/nist-janaf-thermochemical-tables-4th-edition, accessed on 2024.
M. F. Mabrook and M. C. Petty, “Effect of Composition on the Electrical Conductance of Milk,” Journal of Food Engineering, vol. 60, no. 3, pp. 321-325, 2003.
O. Simsek and S. S. Seker, “Forensic Discrimination of Blue Fountain Pen Inks Based on Dielectric Constant Property,” Discover Applied Sciences, vol. 7, article no. 21, 2025.
P. K. Tewari, R. K. Verma, M. P. S. Ramani, and S. P. Mahajan, “Studies on Nucleate Boiling of Sodium Chloride Solutions at Atmospheric and Sub-Atmospheric Pressures,” Desalination, vol. 52, no. 3, pp. 335-344, 1985.
J. Gu, Y. Wu, G. Tang, Q. Wang, and J. Lyu, “Experimental Study of Heat Transfer and Bubble Behaviors of NaCl Solutions During Nucleate Flow Boiling,” Experimental Thermal and Fluid Science, vol. 109, article no. 109907, 2019.
T. Xu and K. Pruess, “Thermophysical Properties of Sodium Nitrate and Sodium Chloride Solutions and Their Effects on Fluid Flow in Unsaturated Media,” Ernest Orlando Lawrence Berkeley National Laboratory, Technical Report LBNL-48913, 2001.
T. K. Bera and J. Nagaraju, “Electrical Impedance Spectroscopic Studies on Broiler Chicken Tissue Suitable for the Development of Practical Phantoms in Multifrequency EIT,” Journal of Electrical Bioimpedance, vol. 2, no. 1, 2011.
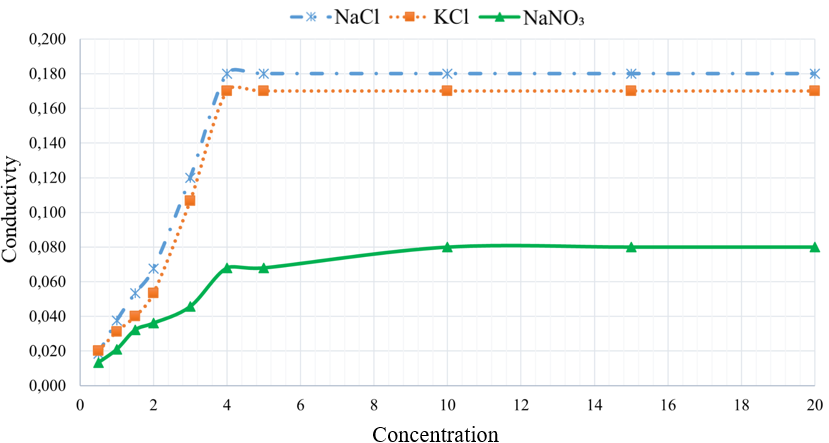
Published
How to Cite
Issue
Section
License
Copyright (c) 2025 Nigar Kantarci-Carsibasi, Jana Masalmah, Ozlem Simsek

This work is licensed under a Creative Commons Attribution-NonCommercial 4.0 International License.
Submission of a manuscript implies: that the work described has not been published before that it is not under consideration for publication elsewhere; that if and when the manuscript is accepted for publication. Authors can retain copyright in their articles with no restrictions. is accepted for publication. Authors can retain copyright of their article with no restrictions.
Since Jan. 01, 2019, AITI will publish new articles with Creative Commons Attribution Non-Commercial License, under The Creative Commons Attribution Non-Commercial 4.0 International (CC BY-NC 4.0) License.
The Creative Commons Attribution Non-Commercial (CC-BY-NC) License permits use, distribution and reproduction in any medium, provided the original work is properly cited and is not used for commercial purposes.